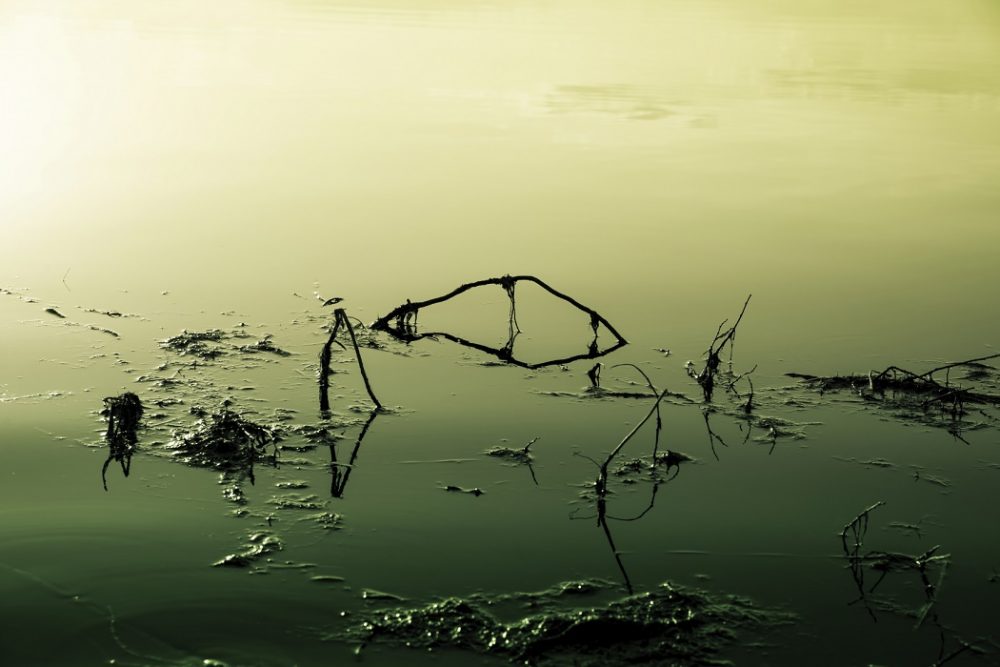
JONATHAN LI – NOVEMBER 9TH, 2022
Abstract
This paper attempts to find the causal effect of phase II of the Acid Rain Program’s on SO2 emissions from US power plants as well as the frequency of acidic precipitation and surface water bodies. I utilized a regression discontinuity model to overcome omitted variable bias in order to accurately measure the program’s impact. I find that phase II of the Acid Rain Program resulted in a statistically significant decrease in SO2 emissions by 1650 tons. However, I fail to find a statistically significant coefficient for acid rain. While there is some moderate evidence that the ARP decreased acidic surface waters, the decrease does not amount to anything environmentally significant.
1. Introduction
1.1 Background
In the early 1980s, scientists observed that lakes and streams across the U.S. were becoming overly acidic, leading to declining populations of fish, amphibians, and other forms of aquatic life that could not bear the sudden rise in acidity. On land, soil nutrients became depleted and many buildings and infrastructure began to weather away. Hence, in 1980, Congress called on the EPA and several other environmental agencies to conduct research on the culprits of acidic rain and its effects (EPA, 2020). The research identified Sulfur Dioxide (SO2) and Nitrogen Oxide (NOx) released from electric power plants as the major contributor to acid rain. This realization resulted in the formation of the Acid Rain Program (ARP) – one of the numerous amendments made to the US Clean Air Act in 1990.
Absent any controls to limit acidic precipitation, aquatic ecosystems and forests will bear severe consequences. Acid rain that seeks into the ground dissolves crucial nutrients, such as calcium and magnesium, that forests rely upon to sustain themselves. In aquatic ecosystems, certain species of fish such as trout and mouth bass cannot tolerate low pH levels, leading to ripple effects in aquatic food webs in surface waters across the country (Nunez, 2019). The effects of acid rain are not confined to environmental damages; pollutants SO2 and NOx have been found to cause respiratory diseases such as asthma and bronchitis, potentially resulting in permanent lung damage.
In the 21st century, acid rain is no longer on the frontline of US environmental concerns, replaced by other major concerns like climate change. Many credit this shift to the ARP, which utilized an economic cap and trade system to force power plants across the US to reduce its SO2 emissions by 10 million tons below 1980 levels of about 18.9 million tons. This objective was accomplished over time in two separate phases. Under phase I, 110 power plants (263 electricity-generating units) had to scale back emissions in order to achieve half of the program’s goal by January 1, 1995. Phase II saw all units over 75MWe limit their SO2 emissions to 1.2 pounds per million by January 1, 2000 in order to fully accomplish the program’s objective. After the completion of phase II, the EPA continued to distribute allowances under a cap and trade system to be able to continuously monitor SO2 emissions across the country (EPA). The goal of this paper is to answer the following questions: how much did the ARP reduce SO2 emissions by? Did the ARP’s reduction of SO2 translate into tangible reductions of overly acidic precipitation and surface water bodies (lakes and rivers)?
1.2 Overview of Literature Body
Previous literature studies on the ARP are generally flawed in one of two ways. First, past studies have attempted to quantify the effects of both phase I and II of the program. Unfortunately, I would make the case that it is impossible to accurately quantify phase 1 of the program. Prior to 1990, the EPA only released data on SO2 emissions once every 5 years (i.e 1975, 1980, 1985, 1990). Due to this lack of annual data, other studies make the assumption that SO2 emissions remain constant from 1990-1994 and conduct a regression discontinuity to quantify the effects of phase 1 (i.e. average of 1994 vs 1995). I believe this assumption is fundamentally flawed; it could be the case that emissions were already on a downward trend prior to the phase 1 deadline (January 1, 1995). Thus, it is impossible to actually pinpoint the causal effect of phase I of the program. Next, previous studies have not attempted to quantify the reduction of acidic rain attributable to the ARP, the entire objective of the program. Even if there is a statistically significant reduction in SO2, it is meaningless unless it amounts into a reduction in acidic rain and surface waters as well. In section 2, I delve deeper into the literature body to analyze where this paper will fit in.
1.3 Research Question
My paper will aim to answer the following closely-related questions. What is the causal effect of phase II of the ARP on SO2 emissions from US power plants? If there is a causal effect on SO2 emissions, did it lead to a significant reduction in overly-acidic precipitation and surface water bodies? Under phase II, electric power plants had to curb their emissions by the Jan 1, 2000 deadline. To extract a causal estimate from the program, I run a regression discontinuity framework to observe if there is a statistically significant difference in our variables of interest from 1999 to 2000. I find that the ARP did indeed result in a statistically significant reduction in SO2 emissions. However, the reduction in SO2 did not translate into a statistically significant reduction in acidic precipitation. My paper also provides moderate evidence (p-value of 9%) that the ARP reduced the prevalence of acidic surface water by 0.0273%. Regardless of whether or not this reduction is statistically significant, this certainly does not amount to anything environmentally significant.
While there are numerous prominent studies related to the Acid Rain Program, none have actually quantified the causal effect of the ARP on acid rain and surface water. Other notable papers in the field have covered topics such as the cost efficiency of the ARP as well as its health effects, but none of them provide a causal estimate of the ARP on acid rain. The objective of my paper is to help fill this gap and lay a foundation for further improved work on this topic.
2. Literature Review
Plenty of academic literature already exists on the ARP and other relevant topics (i.e. the environmental consequences of air pollutants). I will conduct a literature review by first providing a summary on the role air pollutants have on human health and mortality rates. Then, I will dive into prominent studies that focus specifically on the efficacy of the ARP. Finally, I will conclude by justifying how this paper will contribute to the literature body.
2.1 Relevant Literature
An abundance of literature exists on the effect of increases in SO2 on human health; however, many of them fail to accurately extract causality. The challenge behind this body of literature is that SO2 is highly correlated with other other harmful pollutants, such as NOx. Therefore, effective studies must have effective control mechanisms to isolate the health effects of SO2. A study conducted in Asian countries used data from Bangkok, Thailand, and three major Chinese cities to construct a poisson regression model found that an increase of 10 μg/m3 of 2-day moving average concentrations of SO2 corresponds to 1% increase of total, cardiovascular, and respiratory mortality (Kan, Wong, Vichi-Vadakan, Qian, 2012). These findings appear to be fairly consistent with other studies; a meta-analysis pooling the results of 1,128 articles found that a 10 μg/m3 increase in SO2 is associated with approximately a 1% gain in all-cause mortality, respiratory mortality with a statistical significance at the 1% level (Orellano, Reynoso, Quaranta, 2021). These results still remained statistically significant even after controlling for PM2.5 particles – other air pollutants that damage people’s health when levels are high. In summation, there is a pretty strong base of documentation to prove that increased SO2 adversely affects human health via increased all-cause and respiratory mortality.
There have been a few notable past studies conducted on the efficacy of the ARP. First off, a 2005 study provides a comprehensive cost-benefit analysis of the acid rain program. The authors find that SO2 and NOx emissions substantially contribute to the formation of additional PM2.5 particles. Hence, the authors then conclude that reductions in emissions results in health benefits that far outweigh the expected costs of the ARP (Chestnut, Mills 2005). Another more recent study conducted in 2021 drawed a similar conclusion; the authors use a differences-in-differences model to compare changes in adult mortality over time driven by installation of sulfur controls on electric units in power plants. They found that pollution from power plants dipped immediately, which translated into reductions in mortality that grew over time, suggesting cumulative health effects (Barreca, Neidell, Sanders, 2021). However, when estimating the size of the pollution decrease from the ARP, the authors of this study make the erroneous assumption I explained earlier in section 1.2. To quantify the causal effect of Phase I SO2 reductions, the authors run a regression discontinuity model as well. Due to limitations in data, they assume that SO2 levels remain constant from 1990-1994. Therefore, when they compare SO2 emissions between 1994 and 1995, they are actually comparing SO2 levels between 1990 and 1995, resulting in a model that I believe to be faulty.
To economically quantify these health effects, a 2003 study from the Office of Management and Budget concluded that the human health benefits from the ARP equates to $70 billion annually over ten years (1993-2003), exceeding costs by a 40:1 ratio (Office of Management and Budget, 2003). Finally, one study compared the cost savings of the ARP to a command and control alternative, where policymakers tightly regulate how factories will scale back on their emissions as opposed to giving factories freedom for how to control their own emissions. The authors compare the costs of the ARP to a counterfactual command and control system that should theoretically achieve the same aggregate emissions reductions. They conclude that the ARP has an annual cost savings of approximately $250 million relative to command and control policies (Chan, Chupp, Cropper, Muller, 2015). Evidently, an abundance of literature already exists on the ARP and similarly-related fields. In the next section, I will argue where this paper fits into the mix.
2.2 Contribution to the Field
To summarize, the body of literature on the effectiveness of the Acid Rain Program is overwhelmingly positive: it did indeed sharply decrease SO2 emissions levels, which translates into benefits on human health, and it is a highly cost-effective system. However, as I alluded to earlier, there is a problematic gap in which the current body of literature does not attempt to pinpoint the effect of SO2 reductions from the ARP onto the frequency of acidic precipitation and surface water. I found this to be particularly odd, as the entire premise of the program is to develop a way to decrease the amount of acid rain in the US. The objective of this paper is to fill this void by providing my own estimate on the causal effect of the ARP on acid rain and surface water.
3. Research Design
Under Phase II of the program, electric power plants were required to scale back their emissions on Jan 1, 2000. Therefore, I utilized a regression discontinuity to compare data points from 1999 to 2000. Comparing points closer together allows me to claim with greater certainty that any change is likely causal, as opposed to spurious correlation. Comparing points from earlier years (i.e. 1996) results in greater potential for omitted variable bias, such as technological efficiency improvements over time. My experiment will entail three separate regression models that will take on the following form.
(1) Yit = β0 – β1*Yearit – β2 *Overit – β3*(Year_hatit*overit) + εit
In this experiment, I have three separate dependent variables of interest. The first is ‘SO2it’ measured in tons, while ‘surfaceit’ and ‘rainit’ are categorical variables that equal to one if the collected sample of rain or surface water can be considered acidic. To contextualize what classifies as “acidic”, I included the following diagram below from the EPA.
Figure 1:pH Scale Classification
Acidic rain generally has a pH level of around 4.2-4.4; therefore, I programmed the variable ‘acidic_rain’ to be a dummy variable that equals one anytime the collected sample of rain has a pH level less than 4.4. Healthy surface water bodies have a pH level from 6.5-8.5; therefore, I set ‘acidic_surface” to be a dummy variable that equals to one if the sample of surface water has a pH level below 6. First, I will test the effect of the program on SO2 in terms of tons emitted. Next, I will examine the effect of the ARP on the average prevalence of acidic rainfall and surface waters. The regression model uses multiple datasets that follow the same units of observation ‘i’ across time ‘t’ (1995-2005).
The regressors remain the same across all three regression discontinuity equations. The first term ‘year’ is the running variable from 1995 to 2005, and ‘Year_hat’ = Year – 1999. The first coefficient on ‘Year’ (β1) gives us the average annual change from 1995 to 1999. The variable of interest ‘over’ is a dummy variable that equals 1 if Year>1999 (2000 or later), meaning that its coefficient is the average difference between 1999 and 2000. This coefficient represents the causal effect of the ARP on the dependent variables. Finally, the variable ‘(Year_hat*Over)’ and its coefficient gives us the slope after the cutoff line (2000-2005). Importantly, our coefficient of interest in this experiment is ‘β2’, which will help us answer the original research questions posited at the beginning of this paper.
4. Overview of Data
This paper relies on three independent time series datasets from different sources to feed into the regression discontinuity model. The next three subsections will provide a brief summary of the data as well as key mean estimates.
4.1 Power Plant Data
The first dataset comes from the EPA’s Air Markets Program. I collected longitudinal data that quantifies the amount of SO2 and NOx from electricity-generating units (EGU) in power plants. The data provides an identification number and location for each individual EGU and tracks their emissions over time. The dataset has a total of 27,993 observations total across 1995-2005; the key summary statistics are features below. For this paper’s purposes, I am only interested in the statistics for SO2 – one of the dependent variables for the regression model.
4.2 Acid Rain Data
The dataset on acid rain originates from the National Atmospheric Decomposition Program (NADP). Across the country, site operators collect weekly samples of the previous week’s precipitation every Tuesday morning, and then the samples are sent to a nearby lab for analysis. From their site, I was able to collect longitudinal data on the weekly samples from 1995-2005, amounting to a total of 2,486 observations. The data provides extensive detail on the chemical composition of the collected samples; for our purposes, we are only interested in the pH of the rainfall. Summary statistics on the dataset are included in the figure below.
At a first glance at the table, the mean pH of collected rain samples from 1995-2005 is 4.937, with a median value of 4.856. Clean rainfall typically has a pH value of 5.6, so these collected samples are surprisingly acidic.
4.3 Surface Water Data
The final dataset – from the EPA LTM Program – collects water samples from 172 lakes and streams from 1980-2018. The samples come from regions in the eastern US that are particularly sensitive to the effects of acid rain. The water is collected on site and then taken back to a lab for chemical analysis on its chemical composition, measuring a wide range of variables like pH and Sulfate Ions. Once again, we will only be focusing on pH to answer the original research questions. Generated summary statistics of the dataset are shown below.
Similar to the collection of rainfall samples, the samples of surface water are also surprisingly acidic. A healthy pH range for surface water is 6.5-8.5. Thus, a mean pH value of 5.7 comes off as a surprise. One potential explanation for this discrepancy is that the collected samples are from eastern regions in the US that are particularly sensitive to acidic precipitation. Hence, it could be the case that the sample I am working with is more acidic than normal surface water bodies across the country.
4.4 Correlational Estimates Over Time
To establish how these estimates have, on average, changed over time during our time frame of interest (1995-2005), I generated results for the following OLS regression equation below. The dependent variables for this equation are SO2 (tons), as well as the pH levels of rainfall (pH_rain) and surface water (pH_surface). I regressed each variable over years to obtain some correlational estimates.
(2) Yit = β0 – β1* Yearit + εit
The results indicate that on average, the average level of SO2 is modestly decreasing over time. The pH levels of precipitation and surface water, while statistically significant due to a large sample size, do not seem to be noticeably changing across time. It is important to note that these estimates are not meant to provide a causal estimate of the ARP, and are just telling a story of what is generally happening to the dependent variables across time. In the line graphs below, I provide a more detailed visualization for how our variables of interest change over a function of time (from 1995-2005).
By taking a quick glance at the line graphs above, it seems to be clear that there is an observable trend with average SO2, where a large dip occurs around the same time of phase II treatment (2000). However, for rainfall and surface water, there is no observable pattern in the data. In the next section, I will focus on extracting a causal relationship between ARP’s phase II treatment and our variables of interest.
5. Results
5.1 Main Findings
The regression discontinuity models of interest are written explicitly below, with ‘SO2’ measured in tons and ‘surface’ and ‘rain’ as both dummy variables that equal one when the sample can be classified as acidic. Using dummy variables to identify acidic samples of rainfall and surface water allows me to test how the prevalence of collected acidic samples changes.
(3) SO2it = β0 – β1*Yearit – β2 *Overit – β3*(Year_hatit*overit) + εi
(4) Surfaceit = β0 – β1*Yearit – β2 *Overit – β3*(Year_hatit*overit) + εi
(5) Rainit = β0 – β1*Yearit – β2 *Overit – β3*(Year_hatit*overit) + εi
5.2 Discussion of Results
The coefficient on ‘Over’ is what provides the causal effect of the ARP, and hence, is my coefficient of interest. For SO2, I receive a large negative coefficient of -1643.72 that is statistically significant at the 1% level. Based on the research design, I can assert that the ARP led to average SO2 emissions to decrease by approximately 1,650 tons. The next question of interest is whether or not this reduction in SO2 translated into a decrease in acidic rain and surface water bodies. The second regression model returns a statistically insignificant coefficient, with a p-value of 0.573. With such a high p-value, this coefficient can be treated as a statistical zero, implying that the ARP did not affect acid precipitation. Finally, the third regression model outputs a slope of -0.0273 with a p-value of 0.096. Statistically speaking, this would provide moderately-strong evidence that the prevalence of acidic surface water decreased by 0.0273%. However, even if this coefficient estimate is statistically significant, such a miniscule coefficient certainly does not likely amount to anything environmentally significant. Hence, I conclude that while phase II of the ARP did result in a noticeable curb on SO2 emissions, aligning with past literature on this topic. However, the reduction in SO2 did not translate into any meaningful reduction in acid rain and surface water
While these results are mixed, it is important to acknowledge certain assumptions and limitations that could diminish the comprehensiveness and accuracy of our coefficient estimates. As I argued earlier, it is infeasible to accurately quantify the Phase I effects of the program, meaning that we are unable to capture the full causal effect of the entire ARP. Second, our regression discontinuity model assumes that the entire causal change occurred from 1999 to 2000. In reality, it is likely that the causal reduction in SO2 occurred over a wider time frame (i.e. 1997-2000), as firms probably took a longer period of time to fully comply with the phase II requirement. This implies that the coefficient obtained from our model is likely an underestimate to the true parameter; however, there is no realistic way for me to know for certain how each electric power plant in the US responded to the phase II mandate deadline, as each firm was left to their own devices to achieve their required reductions. Next, there may be limitations and faults in the datasets as well. For instance, there were numerous entries left blank (null spaces) in all three of my datasets. Therefore, many of the collected data entries observed over time may have dropped out of the data before the completion of our timeframe of interest (1995-2005). Additionally, the sample collections of surface water were only conducted in certain regions in the U.S., which raises concerns over whether or not the sample is entirely representative of surface water across the country. This last concern, however, is unlikely to be a major issue because the EPA specifically chose regions that tend to be more sensitive to the effects of acid rain.
The largest concern of my entire study is that I potentially made false assumptions about how acid rain responds to a reduction in SO2. In my model, I was able to clearly identify a significant, discontinuous drop off in SO2 emissions from 1999-2000. It could be the case that it takes a longer time for acid rain to respond. For example, a hypothetical drop in SO2 from 1999-2000 may not immediately result in a decrease in acid rain across the same time period. Perhaps, it may take a few years to witness a corresponding drop off in acid rain. This might explain why the coefficient on ‘(Year_hat*Over)’ for acid rain, which represents the average change in acid rain from 2000-2005, returns a highly statistically significant (p<0.01) negative coefficient. Future studies on this topic may demand more creative research designs than a regression discontinuity model to be able to extract the ARP’s causal effect on acidic rainfall.
6. Conclusion
In the early 1980s, acidic rain emerged as a massive environmental challenge in western countries. While acidic precipitation still exists, its prevalence is significantly less than forty years ago. I attempted to measure the reduction in SO2 and acidic rain precipitation and surface water bodies specifically from the ARP, something that has not been done yet by previous studies. I utilized three separate datasets to generate longitudinal data on SO2 emissions from electricity generating units and pH levels from collected samples of rainfall and surface water bodies. I plugged in the data into a regression discontinuity framework that emphasizes the change in our outcomes of interest – SO2 and pH levels – from 1999 to 2000 to extract a causal estimate. My results indicate that while there was a significant drop in SO2 emissions levels, there was not a reduction in acidic precipitation and surface water, contrary to what I would have initially expected.
References
“Acid Rain Program.” Epa.gov, Environmental Protection Agency, 2019, https://www.epa.gov/acidrain/acid-rain-program.
Barreca, Alan I., et al. “Long-Run Pollution Exposure and Mortality: Evidence from the Acid Rain Program.” Journal of Public Economics, vol. 200, 2021, p. 104440., https://doi.org/10.1016/j.jpubeco.2021.104440.
Chan, Ron, et al. “The Net Benefits of the Acid Rain Program: What Can We Learn from the Grand Policy Experiment?” Regulatory Performance Initiative , June 2015.
Chestnut, Lauraine G., and David M. Mills. “A Fresh Look at the Benefits and Costs of the US Acid Rain Program.” Journal of Environmental Management, vol. 77, no. 3, 2005, pp. 252–266., https://doi.org/10.1016/j.jenvman.2005.05.014.
Kan, Haidong, et al. “Short-Term Association between Sulfur Dioxide and Daily Mortality: The Public Health and Air Pollution in Asia (PAPA) Study.” Environmental Research, vol. 110, no. 3, 2010, pp. 258–264., https://doi.org/10.1016/j.envres.2010.01.006.
Nunez, Christina. “Acid Rain, Explained.” Nationalgeographic.com, National Geographic, 4 May 2021, https://www.nationalgeographic.com/environment/article/acid-rain.
Orellano, Pablo, et al. “Short-Term Exposure to Sulphur Dioxide (SO2) and All-Cause and Respiratory Mortality: A Systematic Review and Meta-Analysis.” Environment International, vol. 150, 2021, p. 106434., https://doi.org/10.1016/j.envint.2021.106434.
“The Legacy of EPA’s Acid Rain Research.” Epa.gov, Environmental Protection Agency, 18 Aug. 2020, https://www.epa.gov/sciencematters/legacy-epas-acid-rain-research#:~:text=Acid%20rain%20negatively%20affects%20aquatic,amphibians%2C%20and%20other%20aquatic%20life.
United States, Congress, Office of Management and Budget. Draft 2003 Report to Congress on the Costs and Benefits of Federal Regulations; Notice, Office of Management and Budget, 2003, pp. 1–37.
Featured Image Source: Daily JSTOR
Disclaimer: The views published in this journal are those of the individual authors or speakers and do not necessarily reflect the position or policy of Berkeley Economic Review staff, the Undergraduate Economics Association, the UC Berkeley Economics Department and faculty, or the University of California, Berkeley in general.